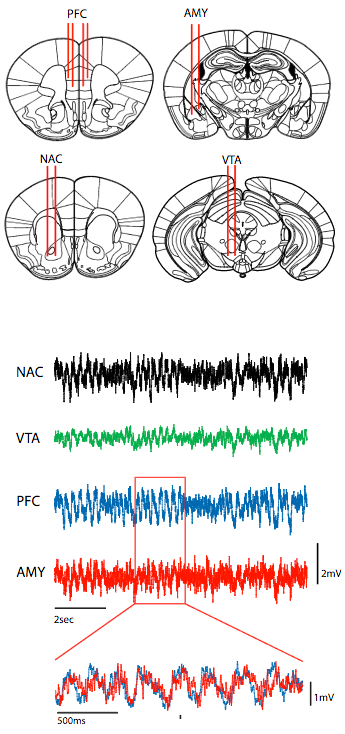
To directly probe the relationship between prefrontal cortex circuitry (PFC), limbic network oscillatory dysfunction, and the emergence of depression-related behavior, the researchers implanted mice with microwire recording electrodes in PFC and three relevant limbic brain regions implicated in major depressive disorder: nucleus accumbens (NAC), amygdala (AMY), and ventral tegmental area (VTA). Shown below: representative local field potential traces of neural signals. In the overlaid traces below, note that PFC oscillations (blue) tended to precede AMY oscillations (red). (credit: Rainbo Hultman et al./Neuron)
Imagine if doctors could precisely insert a tiny amount of a custom drug into a specific circuit in your brain and improve your depression (or other mood problems) — instead of treating the entire brain.
That’s exactly what Duke University researchers have explored in mice. Stress-susceptible animals that appeared depressed or anxious were restored to relatively normal behavior this way, according to a study appearing in the forthcoming July 20 issue of Neuron.
The plan was to define specific glitches in the neural circuits and then use a drug to fix them. The ambitious goal: go from a protein, to a signaling activity, to a cell, to a circuit, to activity that happens across the whole brain, to actual behavior.
1. Identify the key neurons in the prefrontal cortex
The researchers first determined how the prefrontal cortex is used as a pacemaker for the limbic system, said lead researcher Kafui Dzirasa, an assistant professor of psychiatry and behavioral sciences, and neurobiology.
The team started by precisely placing arrays of 32 electrodes in four brain areas of the mice (see illustration above). Then they recorded brain activity as these mice were subjected to a stressful situation called chronic social defeat.* This allowed the researchers to observe the activity between the prefrontal cortex and three areas of the limbic system that are implicated in major depression.
To interpret the complicated data coming from the electrodes, the team used machine learning algorithms — identifying which parts of the data seemed to be the timing control signal between the prefrontal cortex and the limbic system— and then zeroed in on the individual neurons involved in that cortical signal and its corresponding circuit.
2. Inject a drug to restore function
They then applied engineered molecules called DREADD (Designer Receptors Exclusively Activated by Designer Drug), developed by University of North Carolina at Chapel Hill pharmacologist Bryan Roth, in very tiny amounts (0.5 microliter). A drug that attaches only to that DREADD is then administered to give the researchers control over the circuit.
They found that direct stimulation of PFC-amygdala neural circuitry with DREADDs normalized PFC-dependent limbic synchrony in stress-susceptible animals and restored normal behavior.
The researchers suggest that their findings also demonstrate an interdisciplinary approach that can be used to identify the large-scale network changes that underlie complex emotional pathologies and the specific network nodes that can be used to develop targeted interventions.
“These subcortical circuits are the key regulators of our emotional life,” said Helen Mayberg, a professor of psychiatry, neurology and radiology at Emory University who was not involved in this research. “What’s great about this paper is that they use different approaches to see a circuit that’s relevant to a lot of disorders,” said Mayberg, who has been pioneering deep-brain stimulation of very specific sites in the human prefrontal cortex to treat mood disorders.
But she cautions that to assess anything like “mood” in a mouse, one can only infer from its behaviors. “It’s hard to do, even in a human,” she said.
This work was supported by funding from National Institutes of Mental Health and a research incubator award from the Duke Institute for Brain Sciences.
* The mice were repeatedly exposed to larger aggressive animals for 10–15 consecutive days. At the end of this protocol, animals exhibit multiple depressive endophenotypes including hedonic dysfunction, circadian dysregulation, anxiety, and psychomotor retardation.
Abstract of Dysregulation of Prefrontal Cortex-Mediated Slow-Evolving Limbic Dynamics Drives Stress-Induced Emotional Pathology
Circuits distributed across cortico-limbic brain regions compose the networks that mediate emotional behavior. The prefrontal cortex (PFC) regulates ultraslow (<1 Hz) dynamics across these networks, and PFC dysfunction is implicated in stress-related illnesses including major depressive disorder (MDD). To uncover the mechanism whereby stress-induced changes in PFC circuitry alter emotional networks to yield pathology, we used a multi-disciplinary approach including in vivo recordings in mice and chronic social defeat stress. Our network model, inferred using machine learning, linked stress-induced behavioral pathology to the capacity of PFC to synchronize amygdala and VTA activity. Direct stimulation of PFC-amygdala circuitry with DREADDs normalized PFC-dependent limbic synchrony in stress-susceptible animals and restored normal behavior. In addition to providing insights into MDD mechanisms, our findings demonstrate an interdisciplinary approach that can be used to identify the large-scale network changes that underlie complex emotional pathologies and the specific network nodes that can be used to develop targeted interventions.