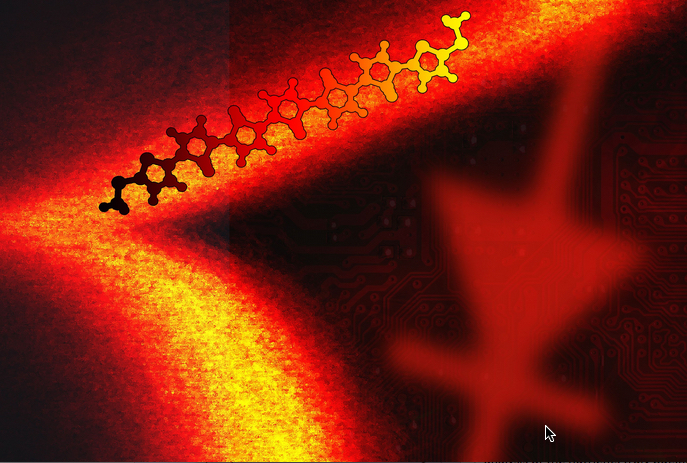
Molecular diode artist’s impression (credit: Columbia Engineering)
Columbia Engineering researchers have created the first single-molecule diode — the ultimate in miniaturization for electronic devices — with potential for real-world applications in electronic systems.
The diode that has a high (>250) rectification and a high “on” current (~ 0.1 microamps), says Latha Venkataraman, associate professor of applied physics. “Constructing a device where the active elements are only a single molecule … which has been the ‘holy grail’ of molecular electronics, represents the ultimate in functional miniaturization that can be achieved for an electronic device,” he said.
With electronic devices becoming smaller every day, the field of molecular electronics has become ever more critical in solving the problem of further miniaturization, and single molecules represent the limit of miniaturization. The idea of creating a single-molecule diode was suggested by Arieh Aviram and Mark Ratner who theorized in 1974 that a molecule could act as a rectifier, a one-way conductor of electric current.
The future of miniaturization
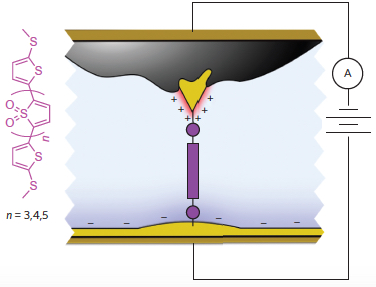
Single-molecule asymmetric molecular structure (alkyl side chains omitted for clarity) using a donor–bridge–acceptor architecture to mimic a semiconductor p–n junction (credit: Brian Capozzi et al./Nature Nanotechnology)
Researchers have since been exploring the charge-transport properties of molecules. They have shown that single-molecules attached to metal electrodes (single-molecule junctions) can be made to act as a variety of circuit elements, including resistors, switches, transistors, and, indeed, diodes.
They have learned that it is possible to see quantum mechanical effects, such as interference, manifest in the conductance properties of molecular junctions.
Since a diode acts as an electricity valve, its structure needs to be asymmetric so that electricity flowing in one direction experiences a different environment than electricity flowing in the other direction. To develop a single-molecule diode, researchers have simply designed molecules that have asymmetric structures.
“While such asymmetric molecules do indeed display some diode-like properties, they are not effective,” explains Brian Capozzi, a PhD student working with Venkataraman and lead author of the paper.
“A well-designed diode should only allow current to flow in one direction … and it should allow a lot of current to flow in that direction. Asymmetric molecular designs have typically suffered from very low current flow in both ‘on’ and ‘off’ directions, and the ratio of current flow in the two has typically been low. Ideally, the ratio of ‘on’ current to ‘off’ current, the rectification ratio, should be very high.”
To overcome the issues associated with asymmetric molecular design, Venkataraman and her colleagues — Chemistry Assistant Professor Luis Campos’ group at Columbia and Jeffrey Neaton’s group at the Molecular Foundry at UC Berkeley — focused on developing an asymmetry in the environment around the molecular junction. They created an environmental asymmetry through a rather simple method: they surrounded the active molecule with an ionic solution and used gold metal electrodes of different sizes to contact the molecule.
Avoiding quantum-mechanical effects
Their results achieved rectification ratios as high as 250 — 50 times higher than earlier designs. The “on” current flow in their devices can be more than 0.1 microamps, which, Venkataraman notes, is a lot of current to be passing through a single-molecule. And, because this new technique is so easily implemented, it can be applied to all nanoscale devices of all types, including those that are made with graphene electrodes.
“It’s amazing to be able to design a molecular circuit, using concepts from chemistry and physics, and have it do something functional,” Venkataraman says. “The length scale is so small that quantum mechanical effects are absolutely a crucial aspect of the device. So it is truly a triumph to be able to create something that you will never be able to physically see and that behaves as intended.”
She and her team are now working on understanding the fundamental physics behind their discovery, and trying to increase the rectification ratios they observed, using new molecular systems.
The study, described in a paper published today (May 25) in Nature Nanotechnology, was funded by the National Science Foundation, the Department of Energy, and the Packard Foundation.