
Colorized scanning electron microscopy (SEM) image of a neuron (orange) interfaced with the nanowire array (green). (credit: Integrated Electronics and Biointerfaces Laboratory, UC San Diego)
A research team* led by engineers at the University of California San Diego has developed nanowire technology that can non-destructively record the electrical activity of neurons in fine detail.
The new technology, published April 10, 2017 in Nano Letters, could one day serve as a platform to screen drugs for neurological diseases and help researchers better understand how single cells communicate in large neuronal networks.
A brain implant
The researchers currently create the neurons in vitro (in the lab) from human induced pluripotent stem cells. But the ultimate goal is to “translate this technology to a device that can be implanted in the brain,” said Shadi Dayeh, PhD, an electrical engineering professor at the UC San Diego Jacobs School of Engineering and the team’s lead investigator.
The technology can uncover details about a neuron’s health, activity, and response to drugs by measuring ion channel currents and changes in the neuron’s intracellular voltage (generated by the difference in ion concentration between the inside and outside of the cell).
The researchers cite five key innovations of this new nanowire-to-neuron technology:
- It’s nondestructive (unlike current methods, which can break the cell membrane and eventually kill the cell).
- It can simultaneously measure voltage changes in multiple neurons and in the future could bridge or repair neurons.**
- It can isolate the electrical signal measured by each individual nanowire, with high sensitivity and high signal-to-noise ratios. Existing techniques are not scalable to 2D and 3D tissue-like structures cultured in vitro, according to Dayeh.
- It can also be used for heart-on-chip drug screening for cardiac diseases.
- The nanowires can integrate with CMOS (computer chip) electronics.***
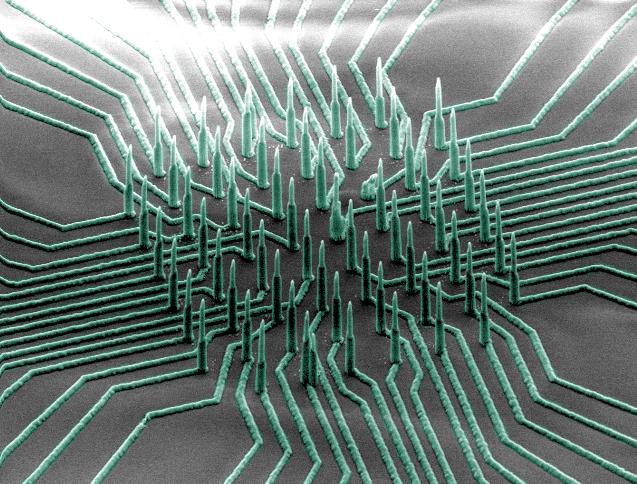
A colorized scanning electron microscopy (SEM) image of the silicon-nickel-titanium nanowire array. The nanowires are densely packed on a small chip that is compatible with CMOS chips. The nanowires poke inside cells without damaging them, and are sensitive enough to measure small voltage changes (millivolt or less). (credit: Integrated Electronics and Biointerfaces Laboratory, UC San Diego)
* The project was a collaborative effort between researchers at UC San Diego, the Conrad Prebys Center for Chemical Genomics at the Sanford Burnham Medical Research Institute, Nanyang Technological University in Singapore, and Sandia National Laboratories. This work was supported by the National Science Foundation, the Center for Brain Activity Mapping at UC San Diego, Qualcomm Institute at UC San Diego, Los Alamos National Laboratory, the National Institutes of Health, the March of Dimes, and UC San Diego Frontiers of Innovation Scholar Program. Dayeh’s laboratory holds several pending patent applications for this technology.
** “Highly parallel in vitro drug screening experiments can be performed using the human-relevant iPSC cell line and without the need of the laborious patch-clamp … which is destructive and unscalable to large neuronal densities and to long recording times, or planar multielectrode arrays that enable long-term recordings but can just measure extracellular potentials and lack the sensitivity to subthreshold potentials. … In vivo targeted modulation of individual neural circuits or even single cells within a network becomes possible, and implications for bridging or repairing networks in neurologically affected regions become within reach.” — Ren Liu et al./Nanoletters
*** The researchers invented a new wafer bonding approach to fuse the silicon nanowires to the nickel electrodes. Their approach involved a process called silicidation, which is a reaction that binds two solids (silicon and another metal) together without melting either material. This process prevents the nickel electrodes from liquidizing, spreading out and shorting adjacent electrode leads. Silicidation is usually used to make contacts to transistors, but this is the first time it is being used to do patterned wafer bonding, Dayeh said. “And since this process is used in semiconductor device fabrication, we can integrate versions of these nanowires with CMOS electronics, but it still needs further optimization for brain-on-chip drug screening.”
Abstract of High Density Individually Addressable Nanowire Arrays Record Intracellular Activity from Primary Rodent and Human Stem Cell Derived Neurons
We report a new hybrid integration scheme that offers for the first time a nanowire-on-lead approach, which enables independent electrical addressability, is scalable, and has superior spatial resolution in vertical nanowire arrays. The fabrication of these nanowire arrays is demonstrated to be scalable down to submicrometer site-to-site spacing and can be combined with standard integrated circuit fabrication technologies. We utilize these arrays to perform electrophysiological recordings from mouse and rat primary neurons and human induced pluripotent stem cell (hiPSC)-derived neurons, which revealed high signal-to-noise ratios and sensitivity to subthreshold postsynaptic potentials (PSPs). We measured electrical activity from rodent neurons from 8 days in vitro (DIV) to 14 DIV and from hiPSC-derived neurons at 6 weeks in vitro post culture with signal amplitudes up to 99 mV. Overall, our platform paves the way for longitudinal electrophysiological experiments on synaptic activity in human iPSC based disease models of neuronal networks, critical for understanding the mechanisms of neurological diseases and for developing drugs to treat them.