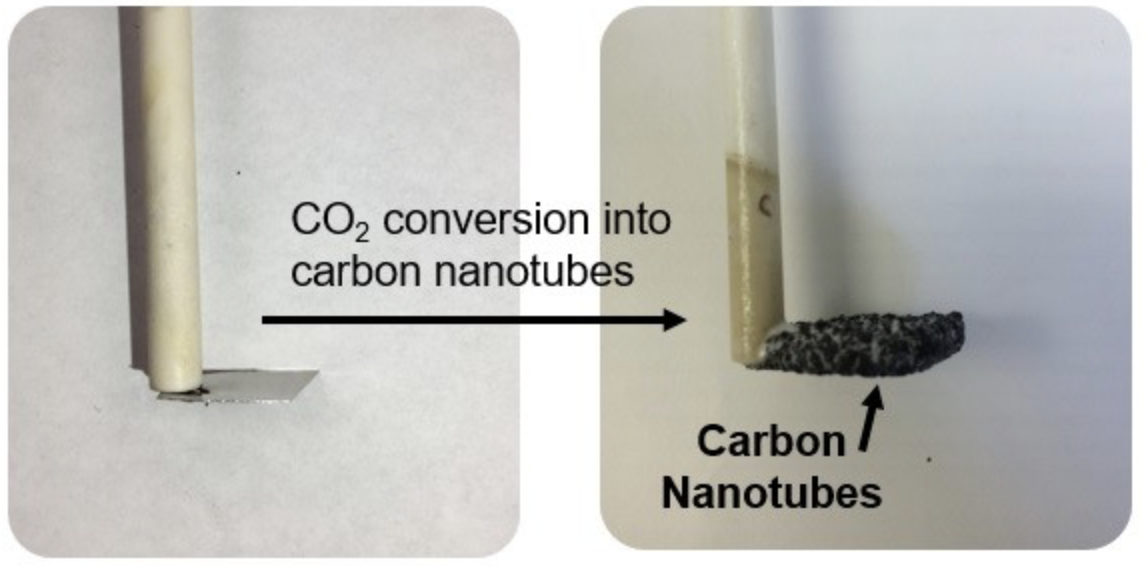
Carbon dioxide converted to small-diameter carbon nanotubes grown on a stainless steel surface. (credit: Pint Lab/Vanderbilt University)
Vanderbilt University researchers have discovered a technique to cost-effectively convert carbon dioxide from the air into a type of carbon nanotubes that they say is “more valuable than any other material ever made.”
Carbon nanotubes are super-materials that can be stronger than steel and more conductive than copper. So despite much research, why aren’t they used in applications ranging from batteries to tires?
Answer: The high manufacturing costs and extremely expensive price, according to the researchers.*
The price ranges from $100–200 per kilogram for the “economy class” carbon nanotubes with larger diameters and poorer properties, up to $100,000 per kilogram and above for the “first class” carbon nanotubes — ones with a single wall, the smallest diameters**, and the most amazing properties, Cary Pint, PhD, an assistant professor in the Mechanical Engineering department at Vanderbilt University, explained to KurzweilAI.
A new process for making cost-effective carbon nanotubes
The researchers have demonstrated a new process for creating carbon-nanotube-based material, using carbon dioxide as a feedstock input source.
- They achieved the smallest-diameter and most valuable CNTs ever reported in the literature for this approach.
- They used sustainable electrochemical synthesis.***
- A spinoff, SkyNano LLC, is now doing this with far less cost and energy input than conventional methods for making these materials. “That means as market prices start to change, our technology will survive and the more expensive technologies will get shaken out of the market,” said Pint. “We’re aggressively working toward scaling this process up in a big way.”
- There are implications for reducing carbon dioxide in the atmosphere.****
“One of the most exciting things about what we’ve done is use electrochemistry to pull apart carbon dioxide into elemental constituents of carbon and oxygen and stitch together, with nanometer precision, those carbon atoms into new forms of matter,” said Pint. “That opens the door to being able to generate really valuable products with carbon nanotubes.” These materials, which Pint calls “black gold,” could steer the conversation from the negative impact of emissions to how we can use them in future technology.
“These could revolutionize the world,” he said.
Reference: ACS Appl. Mater. Interfaces May 1, 2018. Source: Vanderbilt University
* This BCC Research market report has a detailed discussion on carbon nanotube costs: Global Markets and Technologies for Carbon Nanotubes. Also see “Energy requirements,” an open-access supplement to the ACS paper.
** “Small-diameter” in this study refers to about 10 nanometers or less. Small-diameter carbon nanotubes include few-walled (about 3–10 walls), double-walled, and single walled carbon nanotubes. These all have higher economic value because of their enhanced physical properties, broader appeal toward applications, and greater difficulty in synthesis compared to their larger-diameter counterparts. “Larger diameter” carbon nanotubes refer to those with outer diameter generally less than 50 nanometers, since after reaching this diameter, these materials lose the value that the properties in small diameter carbon nanotubes enable for applications.
*** The researchers used mechanisms for controlling electrochemical synthesis of CNTs from the capture and conversion of ambient CO2 in molten salts. Iron catalyst layers are deposited at different thicknesses onto stainless steel to produce cathodes, and atomic layer deposition of Al2O3 (aluminum oxide) is performed on nickel to produce a corrosion-resistant anode. The research team showed that a process called “Ostwald ripening” — where the nanoparticles that grow the carbon nanotubes change in size to larger diameters — is a key contender against producing the infinitely more useful size. The team showed they could partially overcome this by tuning electrochemical parameters to minimize these pesky large nanoparticles.
**** “According to the EPA, the United States alone emits more than 6,000 million metric tons of carbon dioxide into the atmosphere every year. Besides being implicated as a contributor to global climate change, these emissions are currently wasted resources that could otherwise be used productively to make useful materials. At SkyNano, we focus on the electrochemical conversion of carbon dioxide into all carbon-based nanomaterials which can be used for a variety of applications. Our technology overcomes cost limitations associated with traditional carbon nanomaterial production and utilizes carbon dioxide as the only direct chemical feedstock.” — SkyNano Technologies