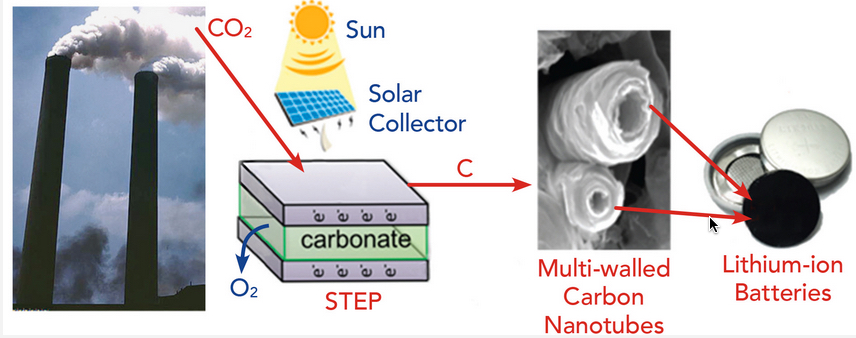
The Solar Thermal Electrochemical Process (STEP) converts atmospheric carbon dioxide into carbon nanotubes that can be used in advanced batteries. (credit: Julie Turner, Vanderbilt University)
The electric vehicle of the future will be carbon negative (reducing the amount of atmospheric carbon dioxide) not just carbon neutral (not adding CO2 to the atmosphere), say researchers at Vanderbilt University and George Washington University (GWU).
The trick: replace graphite electrodes in lithium-ion batteries (used in electric vehicles) with carbon nanotubes and carbon nanofibers recovered from carbon dioxide in the atmosphere. The new technology could also be used in sodium-ion batteries, currently under development for large-scale applications, such as the electric grid.
How to convert CO2 to carbon nanotubes
As described in an open-access paper in the Mar. 2 issue of the journal ACS Central Science, the project builds on a solar thermal electrochemical process (STEP) that can create carbon nanofibers from ambient carbon dioxide (see “‘Diamonds from the sky’ approach to turn CO2 into valuable carbon nanofibers“).
STEP uses solar energy to provide both the electrical and thermal energy needed to break down carbon dioxide into carbon and oxygen and to produce carbon nanotubes, which are stable, flexible, conductive and stronger than steel.
In lithium-ion batteries, the nanotubes replace the carbon anode used in commercial batteries. The team demonstrated that the carbon nanotubes gave a small boost to the performance, which was amplified when the battery was charged quickly.
In sodium-ion batteries, the researchers found that small defects in the carbon, which can be tuned using STEP, can unlock stable storage performance more than 3.5 times above that of sodium-ion batteries with graphite electrodes.
Both carbon-nanotube batteries were exposed to about 2.5 months of continuous charging and discharging and showed no sign of fatigue.
Depending on the specifications, making one of the two electrodes out of carbon nanotubes means that up to 40 percent of a battery could be made out of recycled CO2, according to Vanderbilt Assistant Professor of Mechanical Engineering Cary Pint, not including packaging (which could also be replaced in the future).
Cost benefits
This approach also reduces end-user battery cost, unlike most efforts to reuse CO2 aimed at low-valued fuels, like methanol, which “cannot justify the cost required to produce them,” Pint said.
“Other applications for the carbon nanotubes include carbon composites for strong, lightweight construction materials, sports equipment and car, truck and airplane bodies,” said GWU Professor of Chemistry Stuart Licht.
The researchers estimate that with a battery cost of $325 per kWh (the average cost of lithium-ion batteries reported by the Department of Energy in 2013), a kilogram of carbon dioxide has a value of about $18 as a battery material — six times more than when it is first converted to methanol — a number that increases when moving from large batteries used in electric vehicles to the smaller batteries used in electronics.
And unlike methanol, combining batteries with solar cells provides renewable power with zero greenhouse emissions.

Comparison of conventional natural-gas plant (A), which has CO2 as an exhaust, with carbon nanofiber/carbon nanotube-based natural-gas plant (B) (steam turbine cooling/electricity-generating process (pink), common to both, omitted) (credit: Stuart Licht et al./ACS Central Science)
Licht also proposed that the STEP process could be coupled to a natural gas-powered electrical generator. The generator would provide electricity, heat, and a concentrated source of carbon dioxide that would boost the performance of the STEP process.
At the same time, the oxygen released in the process could be piped back to the generator, where it would boost the generator’s combustion efficiency to compensate for the amount of electricity that the STEP process consumes. The end result could be a fossil fuel electrical power plant with net-zero CO2 emissions.
The research was partially supported by National Science Foundation and NSF Graduate Research Fellowship grants.
Abstract of Carbon Nanotubes Produced from Ambient Carbon Dioxide for Environmentally Sustainable Lithium-Ion and Sodium-Ion Battery Anodes
The cost and practicality of greenhouse gas removal processes, which are critical for environmental sustainability, pivot on high-value secondary applications derived from carbon capture and conversion techniques. Using the solar thermal electrochemical process (STEP), ambient CO2 captured in molten lithiated carbonates leads to the production of carbon nanofibers (CNFs) and carbon nanotubes (CNTs) at high yield through electrolysis using inexpensive steel electrodes. These low-cost CO2-derived CNTs and CNFs are demonstrated as high performance energy storage materials in both lithium-ion and sodium-ion batteries. Owing to synthetic control of sp3 content in the synthesized nanostructures, optimized storage capacities are measured over 370 mAh g–1 (lithium) and 130 mAh g–1 (sodium) with no capacity fade under durability tests up to 200 and 600 cycles, respectively. This work demonstrates that ambient CO2, considered as an environmental pollutant, can be attributed economic value in grid-scale and portable energy storage systems with STEP scale-up practicality in the context of combined cycle natural gas electric power generation.