
A future robot’s body could combine soft actuators and stiff structure, with distributed computation throughout — an example of the new “material robotics.” (credit: Nikolaus Correll/University of Colorado)
Future robots won’t be limited to humanoid form (like Boston Robotics’ formidable backflipping Atlas). They’ll be invisibly embedded everywhere in common objects.
Such as a shoe that can intelligently support your gait, change stiffness as you’re running or walking, and adapt to different surfaces — or even help you do backflips.
That’s the vision of researchers at Oregon State University, the University of Colorado, Yale University, and École Polytechnique Fédérale de Lausanne, who describe the burgeoning new field of “material robotics” in a perspective article published Nov. 29, 2017 in Science Robotics. (The article cites nine articles in this special issue, three of which you can access for free.)
Disappearing into the background of everyday life
The authors challenge a widespread basic assumption: that robots are either “machines that run bits of code” or “software ‘bots’ interacting with the world through a physical instrument.”
“We take a third path: one that imbues intelligence into the very matter of a robot,” says Oregon State University researcher Yiğit Mengüç, an assistant professor of mechanical engineering in OSU’s College of Engineering and part of the college’s Collaborative Robotics and Intelligent Systems Institute.
On that path, materials scientists are developing new bulk materials with the inherent multifunctionality required for robotic applications, while roboticists are working on new material systems with tightly integrated components, disappearing into the background of everyday life. “The spectrum of possible approaches spans from soft grippers with zero knowledge and zero feedback all the way to humanoids with full knowledge and full feedback,” the authors note in the paper.
For example, “In the future, your smartphone may be made from stretchable, foldable material so there’s no danger of it shattering,” says Mengüç. “Or it might have some actuation, where it changes shape in your hand to help with the display, or it can be able to communicate something about what you’re observing on the screen. All these bits and pieces of technology that we take for granted in life will be living, physically responsive things, moving, changing shape in response to our needs, not just flat, static screens.”
Soft robots get superpowers

Origami-inspired artificial muscles capable of lifting up to 1,000 times their own weight, simply by applying air or water pressure (credit: Shuguang Li/Wyss Institute at Harvard University)
As a good example of material-enabled robotics, researchers at the Wyss Institute at Harvard University and MIT’s Computer Science and Artificial Intelligence Laboratory (CSAIL) have developed origami-inspired, programmable, super-strong artificial muscles that will allow future soft robots to lift objects that are up to 1,000 times their own weight — using only air or water pressure.
The actuators are “programmed” by the structural design itself. The skeleton folds define how the whole structure moves — no control system required.
That allows the muscles to be very compact and simple, which makes them more appropriate for mobile or body-mounted systems that can’t accommodate large or heavy machinery, says Shuguang Li, Ph.D., a Postdoctoral Fellow at the Wyss Institute and MIT CSAIL and first author of an an open-access article on the research published Nov. 21, 2017 in Proceedings of the National Academy of Sciences (PNAS).
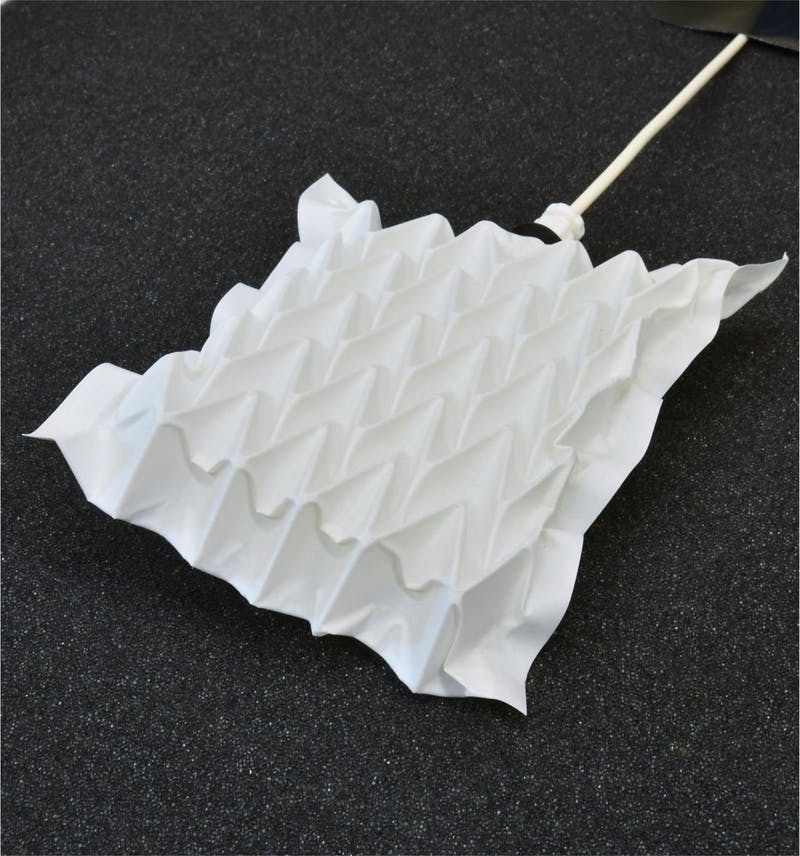
Each artificial muscle consists of an inner “skeleton” that can be made of various materials, such as a metal coil or a sheet of plastic folded into a certain pattern, surrounded by air or fluid and sealed inside a plastic or textile bag that serves as the “skin.” The structural geometry of the skeleton itself determines the muscle’s motion. A vacuum applied to the inside of the bag initiates the muscle’s movement by causing the skin to collapse onto the skeleton, creating tension that drives the motion. Incredibly, no other power source or human input is required to direct the muscle’s movement — it’s automagically determined entirely by the shape and composition of the skeleton. (credit: Shuguang Li/Wyss Institute at Harvard University)
Resilient, multipurpose, scalable
Not only can the artificial muscles move in many ways, they do so with impressive resilience. They can generate about six times more force per unit area than mammalian skeletal muscle can, and are also incredibly lightweight. A 2.6-gram muscle can lift a 3-kilogram object, which is the equivalent of a mallard duck lifting a car. Additionally, a single muscle can be constructed within ten minutes using materials that cost less than $1, making them cheap and easy to test and iterate.
These muscles can be powered by a vacuum, which makes them safer than most of the other artificial muscles currently being tested. The muscles have been built in sizes ranging from a few millimeters up to a meter. So the muscles can be used in numerous applications at multiple scales, from miniature surgical devices to wearable robotic exoskeletons, transformable architecture, and deep-sea manipulators for research or construction, up to large deployable structures for space exploration.
The team could also construct the muscles out of the water-soluble polymer PVA. That opens the possibility of bio-friendly robots that can perform tasks in natural settings with minimal environmental impact, or ingestible robots that move to the proper place in the body and then dissolve to release a drug.
The team constructed dozens of muscles using materials ranging from metal springs to packing foam to sheets of plastic, and experimented with different skeleton shapes to create muscles that can contract down to 10% of their original size, lift a delicate flower off the ground, and twist into a coil, all simply by sucking the air out of them.
This research was funded by the Defense Advanced Research Projects Agency (DARPA), the National Science Foundation (NSF), and the Wyss Institute for Biologically Inspired Engineering.
Wyss Institute | Origami-Inspired Artificial Muscles
Abstract of Fluid-driven origami-inspired artificial muscles
Artificial muscles hold promise for safe and powerful actuation for myriad common machines and robots. However, the design, fabrication, and implementation of artificial muscles are often limited by their material costs, operating principle, scalability, and single-degree-of-freedom contractile actuation motions. Here we propose an architecture for fluid-driven origami-inspired artificial muscles. This concept requires only a compressible skeleton, a flexible skin, and a fluid medium. A mechanical model is developed to explain the interaction of the three components. A fabrication method is introduced to rapidly manufacture low-cost artificial muscles using various materials and at multiple scales. The artificial muscles can be programed to achieve multiaxial motions including contraction, bending, and torsion. These motions can be aggregated into systems with multiple degrees of freedom, which are able to produce controllable motions at different rates. Our artificial muscles can be driven by fluids at negative pressures (relative to ambient). This feature makes actuation safer than most other fluidic artificial muscles that operate with positive pressures. Experiments reveal that these muscles can contract over 90% of their initial lengths, generate stresses of ∼600 kPa, and produce peak power densities over 2 kW/kg—all equal to, or in excess of, natural muscle. This architecture for artificial muscles opens the door to rapid design and low-cost fabrication of actuation systems for numerous applications at multiple scales, ranging from miniature medical devices to wearable robotic exoskeletons to large deployable structures for space exploration.