
A top view of GrBP5 nanowires on a 2-D surface of graphene (credit: Mehmet Sarikaya/Scientific Reports)
Engineers at the University of Washington have created genetically engineered peptides that self-assemble into arrays of nanowires on two-dimensional nanosheets (single-layer graphene and molybdenum disulfide) to relay information across a bio-nano interface — a first step towards fully self-assembled future biomedical and electro-optical bionanoelectronic devices.
Arrays of peptides could provide organized scaffolds for functional biomolecules, enabling nanoscale bioelectronics interfaces. And designed peptides could be incorporated with metal ions or nanoparticles with specific physical characteristics, thus fine-tuning 2D device performance for chemical and biological sensors.
A bridge between biology and technology
“Bridging this divide would be the key to building the genetically engineered biomolecular solid-state devices of the future,” said UW professor Mehmet Sarikaya in the Departments of Materials Science & Engineering, senior author of an open-access paper published Sept. 22 in Scientific Reports.
The UW team is also planning to develop genetically engineered peptides with specific chemical and structural properties. Their ideal peptide would change the physical properties of synthetic materials and respond to that change. That way, it would transmit “information” from the synthetic material to other biomolecules — bridging the chemical divide between biology and technology.
The peptides function through molecular recognition — the same principles that underlie biochemical interactions such as an antibody binding to its specific antigen or protein binding to DNA.

A schematic showing GrBP5 peptide self-organization with a series of surface processes on graphene: binding, diffusion and self-organization (credit: Yuhei Hayamizu et al./Scientific Reports)
In exploring the properties of 80 genetically selected peptides — which are not found in nature but have the same chemical components as peptides in all proteins — the researchers discovered that one peptide, GrBP5, showed promising interactions with the semimetal graphene. They tested GrBP5’s interactions with several other 2-D nanomaterials that “could serve as the metals or semiconductors of the future,” Sarikaya said.
Their experiments revealed that GrBP5 spontaneously organized into ordered nanowire patterns on graphene. With a few mutations, GrBP5 also altered the electrical conductivity of a graphene-based device, the first step toward transmitting electrical information from graphene to cells via peptides.
New bio-optoelectronic devices
Sarikaya’s team also modified GrBP5 to produce similar results on semiconductor material molybdenum disulfide (MoS2) and other materials* by converting a chemical signal to an optical signal. And they computationally predicted how different arrangements of GrBP5 nanowires would affect the electrical conduction or optical signal properties of each material.
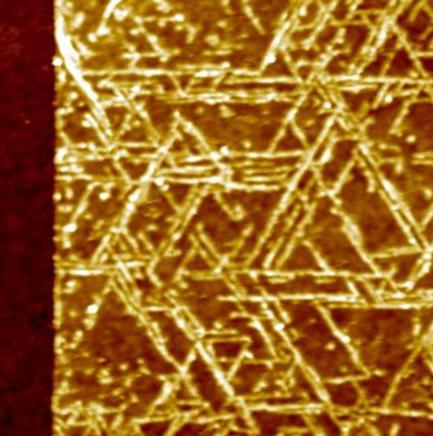
A top view image of GrBP5 nanowires on a 2-D surface of molybdenum disulfide (credit: Mehmet Sarikaya/Scientific Reports)
The researchers are also seeking a peptide that could interact with materials such as gold, titanium, and even a mineral in bone and teeth.
Funded by the National Science Foundation, the UW, the National Institutes of Health, and the Japan Science and Technology Agency, the research is the focus of a new endeavor funded by the National Science Foundation’s Materials Genome Initiative. UW’s CoMotion is also working with Amazon to develop nano-sensors to detect early stages of pancreatic cancer.
* Other semiconducting 2D transition metal dichalcogenides (WSe2, WS2, MoSe2) along with insulating hBN, all with unique electronic and optical properties, were also tested.
Abstract of Bioelectronic interfaces by spontaneously organized peptides on 2D atomic single layer materials
Self-assembly of biological molecules on solid materials is central to the “bottom-up” approach to directly integrate biology with electronics. Inspired by biology, exquisite biomolecular nanoarchitectures have been formed on solid surfaces. We demonstrate that a combinatorially-selected dodecapeptide and its variants self-assemble into peptide nanowires on two-dimensional nanosheets, single-layer graphene and MoS2. The abrupt boundaries of nanowires create electronic junctions via spatial biomolecular doping of graphene and manifest themselves as a self-assembled electronic network. Furthermore, designed peptides form nanowires on single-layer MoS2 modifying both its electric conductivity and photoluminescence. The biomolecular doping of nanosheets defined by peptide nanostructures may represent the crucial first step in integrating biology with nano-electronics towards realizing fully self-assembled bionanoelectronic devices.