
A test cell for the National Spherical Torus Experiment Upgrade with tokamak in the center. (credit: Elle Starkman/PPPL Office of Communications)
Physicists at the U.S. Department of Energy’s Princeton Plasma Physics Laboratory (PPPL)* are building a “star in a jar” — a miniature version of the how our Sun creates energy through fusion. It could provide humankind with near limitless energy, ending dependence on fossil fuels for generating electricity — without contributing greenhouse gases that warm the Earth, and with no long-term radioactive waste.
But that requires a “jar” that can contain superhot plasma — and is low-cost enough to be built around the world. A model for such a “jar,” or fusion device, already exists in experimental form: the tokamak, or fusion reactor. Invented in the 1950s by Soviet physicists, it’s a device that uses a powerful magnetic field to confine plasma (superhot charged gas) in the shape of a torus.
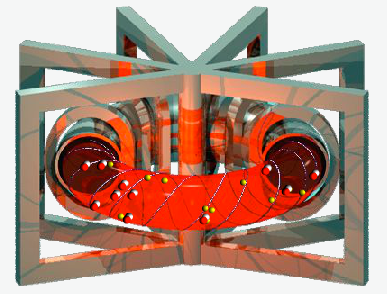
Diagram of magnetic confinement in a tokamak; interactive version: http://www.ccfe.ac.uk/tokamak.aspx (credit: EUROfusion)
There are many experimental tokamaks currently in operation, but they all face physics challenges, the PPPL physicists explain. “For example, they must control the turbulence that arises when superhot plasma particles are subjected to powerful electromagnetic fields. They must also carefully control how the plasma particles interact with the surrounding walls to avoid possible disruptions that can halt fusion reactions if the plasma becomes too dense or impure.”
Spherical tokamak: a new, compact “jar”

Spherical torus/tokamak design for a fusion nuclear science facility showing magnets and other systems and structures (credit: J.E. Menard et al./Nucl. Fusion)
So researchers at PPPL and in and Culham, England are looking at ways of solving these challenges for the next generation of fusion devices, based on compact spherical tokamaks. They suggest that these could provide the design for possible next steps in fusion energy: a Fusion Nuclear Science Facility (FNSF) that would develop reactor components and also produce electricity as a pilot plant for a commercial fusion power station.
The detailed proposal for such a “jar” is described in a paper published in August 2016 in the journal Nuclear Fusion. “We are opening up new options for future plants,” said lead author Jonathan Menard, program director for the recently completed National Spherical Torus Experiment-Upgrade (NSTX-U) at PPPL. The $94-million upgrade of the NSTX, financed by the U.S. Department of Energy’s Office of Science, began operating last year.
Spherical tokamaks are compact devices shaped like cored apples, compared with the bulkier doughnut-like shape of conventional tokamaks. The increased power of the upgraded PPPL machine and the soon-to-be completed MAST Upgrade device “will push the physics frontier, expand our knowledge of high temperature plasmas, and, if successful, lay the scientific foundation for fusion development paths based on more compact designs,” said PPPL Director Stewart Prager.
Next steps in fusion energy
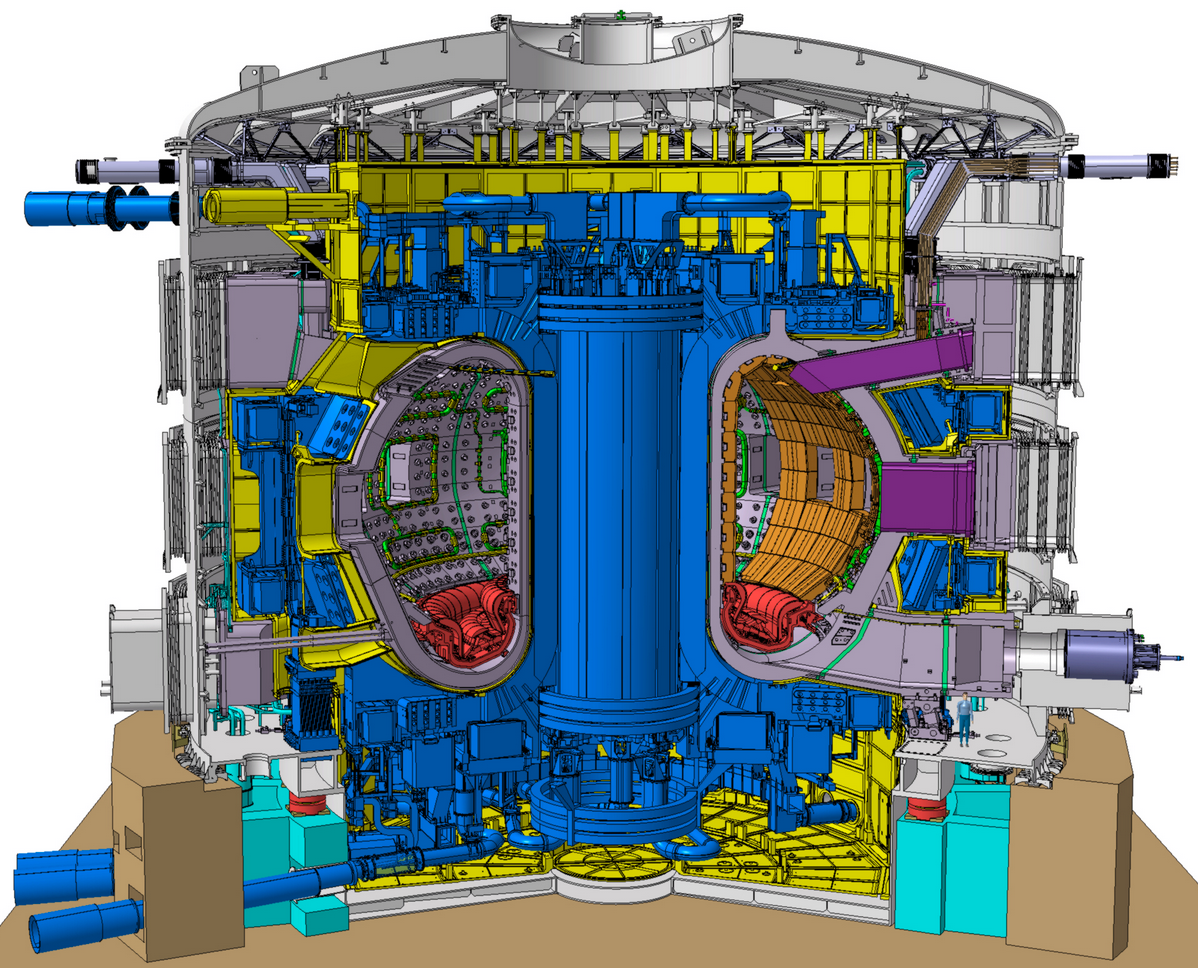
ITER design (credit: ITER Organization)
The spherical design produces high-pressure plasmas — the superhot charged gas (also known as the “fourth state of matter”) that fuels fusion reactions, using relatively low, inexpensive magnetic fields. This unique capability points the way to a possible next generation of fusion experiments to complement ITER, the international tokamak that 35 nations including the U.S. are building in France to demonstrate the feasibility of fusion power. ITER will be the largest in the world when completed within the next decade.
But ITER is a doughnut-shaped tokamak. “The main reason we research spherical tokamaks is to find a way to produce fusion at much less cost than conventional tokamaks require,” said Ian Chapman, the newly appointed chief executive of the United Kingdom Atomic Energy Authority and leader of the UK’s magnetic confinement fusion research programme at the Culham Science Centre.
The 43-page Nuclear Fusion paper describes how the spherical design can provide the next steps in fusion energy. A key issue is the size of the hole in the center of the tokamak, which holds and shapes the plasma. In spherical tokamaks, this hole can be half the size of the hole in conventional tokamaks, and that enables control of the plasma with relatively low magnetic fields.
The smaller hole could also be compatible with a blanket system for the FNSF that would breed tritium, a rare isotope of hydrogen. Tritium will fuse with deuterium, another isotope of hydrogen, to produce fusion reactions in next-step tokamaks.
Superconducting magnets for pilot plants
For pilot FNSF plants, the authors call for superconducting magnets to replace the primary copper magnets in the FNSF. Superconducting magnets can be operated far more efficiently than copper magnets, but require thicker shielding. However, recent advances in high-temperature superconductors could lead to much thinner superconducting magnets that would require less space and reduce considerably the size and cost of the machine.
Included in the paper is a description of a device called a “neutral beam injector” that will start and sustain plasma current without relying on a heating coil in the center of the tokamak. Such a coil is not suitable for continuous long-term operation. The neutral beam injector will pump fast-moving neutral atoms into the plasma and will help optimize the magnetic field that confines and controls the superhot gas.
The researchers believe the upgraded NSTX and MAST facilities will provide crucial data for determining the best path for ultimately generating electricity from fusion. The research is funded by the U.S. Department of Energy.
* PPPL, on Princeton University’s Forrestal Campus in Plainsboro, N.J., is devoted to creating new knowledge about the physics of plasmas and to developing practical solutions for the creation of fusion energy. Results of PPPL research have ranged from a portable nuclear materials detector for anti-terrorist use to universally employed computer codes for analyzing and predicting the outcome of fusion experiments. The Laboratory is managed by the University for the U.S. Department of Energy’s Office of Science, which is the largest single supporter of basic research in the physical sciences in the U.S.
Abstract of Fusion nuclear science facilities and pilot plants based on the spherical tokamak
A fusion nuclear science facility (FNSF) could play an important role in the development of fusion energy by providing the nuclear environment needed to develop fusion materials and components. The spherical torus/tokamak (ST) is a leading candidate for an FNSF due to its potentially high neutron wall loading and modular configuration. A key consideration for the choice of FNSF configuration is the range of achievable missions as a function of device size. Possible missions include: providing high neutron wall loading and fluence, demonstrating tritium self-sufficiency, and demonstrating electrical self-sufficiency. All of these missions must also be compatible with a viable divertor, first-wall, and blanket solution. ST-FNSF configurations have been developed simultaneously incorporating for the first time: (1) a blanket system capable of tritium breeding ratio TBR ≈ 1, (2) a poloidal field coil set supporting high elongation and triangularity for a range of internal inductance and normalized beta values consistent with NSTX/NSTX-U previous/planned operation, (3) a long-legged divertor analogous to the MAST-U divertor which substantially reduces projected peak divertor heat-flux and has all outboard poloidal field coils outside the vacuum chamber and superconducting to reduce power consumption, and (4) a vertical maintenance scheme in which blanket structures and the centerstack can be removed independently. Progress in these ST-FNSF missions versus configuration studies including dependence on plasma major radius