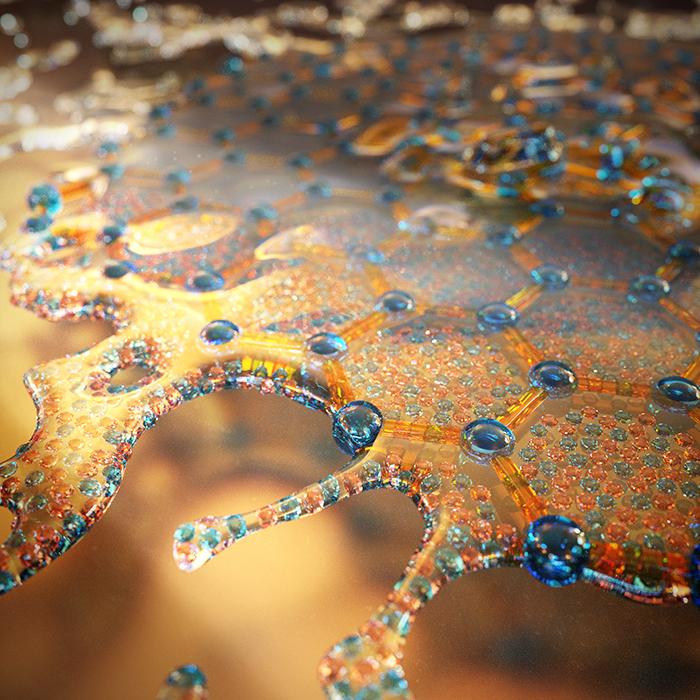
In a new paper published in Science, researchers at the Harvard and Raytheon BBN Technology have observed, for the first time, electrons in a metal behaving like a fluid (credit: Peter Allen/Harvard SEAS)
A radical discovery by researchers at Harvard and Raytheon BBN Technology about graphene’s hidden properties could lead to a model system to explore exotic phenomena like black holes and high-energy plasmas, as well as novel thermoelectric devices.
In a paper published Feb. 11 in Science, the researchers document their discovery of electrons in graphene behaving like a fluid. To make this observation, the team improved methods to create ultra-clean graphene* and developed a new way to measure its thermal conductivity.
A black hole on a chip
In ordinary 3D metals, electrons hardly interact with each other. But graphene’s two-dimensional, honeycomb structure acts like an electron superhighway in which all the particles have to travel in the same lane. The electrons in this ultra-clean graphene act like massless relativistic objects, some with positive charge and some with negative charge.
They move at incredible speed — 1/300 of the speed of light — and have been predicted to collide with each other ten trillion times a second at room temperature. These intense interactions between charge particles have never been observed in an ordinary metal before.
Most of our world is described by classical physics. But very small things, like electrons, are described by quantum mechanics while very large and very fast things, like galaxies, are described by relativistic physics, pioneered by Albert Einstein.
Combining these different sets of laws of physics is notoriously difficult, but there are extreme examples where they overlap. High-energy systems like supernovas and black holes can be described by linking classical theories of hydrodynamics with Einstein’s theories of relativity.
A quantum ‘Dirac’ fluid metal
But since we can’t run an experiment on a black hole (yet), enter graphene.
When the strongly interacting particles in graphene were driven by an electric field, they behaved not like individual particles but like a fluid that could be described by hydrodynamics.
“Physics we discovered by studying black holes and string theory, we’re seeing in graphene,” said Andrew Lucas, co-author and graduate student with Subir Sachdev, the Herchel Smith Professor of Physics at Harvard. “This is the first model system of relativistic hydrodynamics in a metal.”
Industrial implications
A small chip of graphene could also be used to model the fluid-like behavior of other high-energy systems.
To observe the hydrodynamic system, the team turned to noise. At finite temperature, the electrons move about randomly: the higher the temperature, the noisier the electrons. By measuring the temperature of the electrons to three decimal points, the team was able to precisely measure the thermal conductivity of the electrons.
“This work provides a new way to control the rate of heat transduction in graphene’s electron system, and as such will be key for energy and sensing-related applications,” said Leonid Levitov, professor of physics at MIT.
“Converting thermal energy into electric currents and vice versa is notoriously hard with ordinary materials,” said Lucas. “But in principle, with a clean sample of graphene there may be no limit to how good a device you could make.”
The research was led by Philip Kim, professor of physics and applied physics at The Harvard John A. Paulson School of Engineering and Applied Sciences (SEAS).
* The team created an ultra-clean sample by sandwiching the one-atom thick graphene sheet between tens of layers of an electrically insulating perfect transparent crystal with a similar atomic structure of graphene.
“If you have a material that’s one atom thick, it’s going to be really affected by its environment,” said Jesse Crossno, a graduate student in the Kim Lab and first author of the paper. “If the graphene is on top of something that’s rough and disordered, it’s going to interfere with how the electrons move. It’s really important to create graphene with no interference from its environment.”
Next, the team set up a kind of thermal soup of positively charged and negatively charged particles on the surface of the graphene, and observed how those particles flowed as thermal and electric currents.
Harvard John A. Paulson School of Engineering and Applied Sciences | How to Make Graphene
Abstract of Observation of the Dirac fluid and the breakdown of the Wiedemann-Franz law in graphene
Interactions between particles in quantum many-body systems can lead to collective behavior described by hydrodynamics. One such system is the electron-hole plasma in graphene near the charge neutrality point, which can form a strongly coupled Dirac fluid. This charge neutral plasma of quasi-relativistic fermions is expected to exhibit a substantial enhancement of the thermal conductivity, thanks to decoupling of charge and heat currents within hydrodynamics. Employing high sensitivity Johnson noise thermometry, we report an order of magnitude increase in the thermal conductivity and the breakdown of the Wiedemann-Franz law in the thermally populated charge neutral plasma in graphene. This result is a signature of the Dirac fluid, and constitutes direct evidence of collective motion in a quantum electronic fluid.