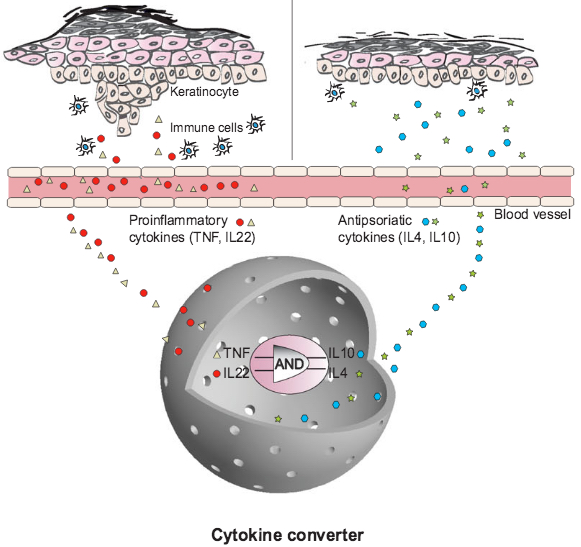
“Cytokine converter” AND-gate synthetic-biology prosthesis used to treat psoriasis in mice. Top left: skin before; right: skin after. (credit: Lina Schukur et al./Science Translational Medicine)
An advanced “molecular prosthetic” — a cell with synthetic gene circuits that can be implanted into an organism to take over metabolic functions that the organism cannot perform itself — has been developed by ETH Zurich scientists.
Previous gene circuits typically monitored only whether one disease-causing molecule (called a cytokine) was present in their environment and if so, produced a single therapeutic cytokine as a response. The new “cytokine converter” synthetic circuit functions like an AND gate: It can detect two different cytokines simultaneously, and if (and only if) both are present, produces two different cytokines that can treat a disease.
As a feasibility study, Professor Martin Fussenegger and his team at ETH’s Department of Biosystems Science and Engineering in Basel used the new cytokine converter prosthesis to treat psoriasis — a complex, chronic inflammatory disease of the skin with no cure — in mice.*
When the cytokine converter detected both of the inflammatory molecules TNF and IL-22, the synthetic gene circuit produced the anti-inflammatory cytokine molecules IL-4 and IL-10, suppressing the inflammatory response.
Preventive treatment for possible wide range of diseases
The symptoms of psoriasis — inflamed, itchy and sometimes flaky areas of skin — are usually combated with a locally applied ointment. “This means that with the existing therapies, we are practically always lagging behind the symptoms,” says Fussenegger.
The gene circuit implant, on the other hand, allowed for prevention: “The circuit begins producing anti-inflammatory messengers at an early stage — when a phase is looming at the level of inflammatory messengers, instead of waiting until skin rashes appear,” he said. It prevented psoriasis “flare-ups” but also treated acute (established) psoriasis, returning skin to normal in mice.
Fussenegger said such molecular prosthetics may one day be implanted in psoriasis patients. However, since growth in connective tissue could cut the implant off from the bloodstream over time, a doctor would probably have to replace it every few months.
Biological circuits with AND gates may also be suitable for other diseases, he said. “Chronic inflammatory diseases are a good example of the type of disease that cannot be diagnosed by measuring a single molecule. However, generally such diseases could be diagnosed using a designer cell that measures the profile of several messengers in the bloodstream. And if this designer cell were also to produce therapeutic molecules, it would open up promising treatment options for a wide range of diseases in the future.”
* Psoriasis is associated with an increased risk of immune-mediated diseases, such as Crohn’s disease and ulcerative colitis, as well as certain cancers (liver and pancreatic), metabolic disorders (obesity and diabetes), and cardiovascular diseases, the authors note.
Abstract of Implantable synthetic cytokine converter cells with AND-gate logic treat experimental psoriasis
Taking pills may go the way of the horse and buggy, the rotary phone, and the Walkman, at least if synthetic biology has anything to say about it. Schukur et al. designed a circuit that would automatically sense the presence of two disease-causing molecules, called cytokines, in the body and respond by triggering the production of two other cytokines that would treat the disease. This circuit was genetically engineered in a mammalian cell; in turn, the cell was implanted in mice with psoriasis—an inflammatory skin condition that has no cure. When levels of the proinflammatory cytokines TNF and IL22 peaked in the body, the synthetic circuit kicked into gear, converting these cytokine signals into an anti-inflammatory cellular output, consisting of IL4 and IL10, which then attenuated disease. The “cytokine converter” cells not only prevented psoriasis flare-ups, as they’re called, but also treated acute (established) psoriasis, returning skin to normal in mice. In demonstrating that the converter cells were responsive to blood from psoriasis patients, the authors suggest that synthetic biology may be ready to autonomously flip therapeutic switches in people and later take on other diseases with defined disease indicators.